Diagnosis of nascent fibrosis
Interest of marking the relaxed form of fibronectin fibres
DOI:
https://doi.org/10.70296/cb-16csqmvns8Keywords:
fibrosis, idiopathic pulmonary fibrosis, PET/CT, biomarker, adhesins, Streptococcus pyogenes, fibronectin, collagens, diagnosis, cancerAbstract
Fibrosis is a pathological and irreversible state of excessively synthesized extracellular matrix fibres induced by abnormal tissue scarring. Effective diagnostic tools for early management of patients with fibrosis are missing. Here, I discuss the recent advances in the quantification of Fibronectin fibres and the interest of novel biologics for diagnosis.
Downloads
References
Wynn, T.A., and Ramalingam, T.R. (2012). Mechanisms of fibrosis: therapeutic translation for fibrotic disease. Nat Med 18, 1028–1040. https://doi.org/10.1038/nm.2807.
Wynn, T.A. (2008). Cellular and molecular mechanisms of fibrosis. Journal of Pathology 214, 199–210. https://doi.org/10.1002/path.2277.
Henderson, N.C., Rieder, F., and Wynn, T.A. (2020). Fibrosis: from mechanisms to medicines. Nature 587, 555–566. https://doi.org/10.1038/s41586-020-2938-9.
Kubow, K.E., Vukmirovic, R., Zhe, L., Klotzsch, E., Smith, M.L., Gourdon, D., Luna, S., and Vogel, V. (2015). Mechanical forces regulate the interactions of fibronectin and collagen i in extracellular matrix. Nature Communications 6, 1–11. https://doi.org/10.1038/ncomms9026.
Gabbiani, G. (2003). The myofibroblast in wound healing and fibrocontractive diseases. Journal of Pathology 200, 500–503. https://doi.org/10.1002/path.1427.
Sidibe, A., Polena, H., Pernet-Gallay, K., Razanajatovo, J., Mannic, T., Chaumontel, N., Bama, S., Marechal, I., Huber, P., Gulino-Debrac, D., et al. (2014). VE-cadherin Y685F knock-in mouse is sensitive to vascular permeability in recurrent angiogenic organs. Am J Physiol Heart Circ Physiol 307, H455-63. https://doi.org/10.1152/ajpheart.00774.2013.
Luo, L., Zhang, W., You, S., Cui, X., Tu, H., Yi, Q., Wu, J., and Liu, O. (2024). The role of epithelial cells in fibrosis: Mechanisms and treatment. Pharmacological Research 202, 107144. https://doi.org/10.1016/j.phrs.2024.107144.
Selman, M., King, T.E., and Pardo, A. (2001). Idiopathic Pulmonary Fibrosis: Prevailing and Evolving Hypotheses about Its Pathogenesis and Implications for Therapy. Ann Intern Med 134, 136. https://doi.org/10.7326/0003-4819-134-2-200101160-00015.
King, T.E., Pardo, A., and Selman, M. (2011). Idiopathic pulmonary fibrosis. The Lancet 378, 1949–1961. https://doi.org/10.1016/S0140-6736(11)60052-4.
Abyaneh, H.S., Regenold, M., McKee, T.D., Allen, C., and Gauthier, M.A. (2020). Towards extracellular matrix normalization for improved treatment of solid tumors. Theranostics 10, 1960–1980. https://doi.org/10.7150/thno.39995.
Teisseire, M., Giuliano, S., and Pagès, G. (2024). Combination of Anti-Angiogenics and Immunotherapies in Renal Cell Carcinoma Show Their Limits: Targeting Fibrosis to Break through the Glass Ceiling? Biomedicines 12, 385. https://doi.org/10.3390/biomedicines12020385.
Watson, S.S., Zomer, A., Fournier, N., Lourenco, J., Quadroni, M., Chryplewicz, A., Nassiri, S., Aubel, P., Avanthay, S., Croci, D., et al. (2024). Fibrotic response to anti-CSF-1R therapy potentiates glioblastoma recurrence. Cancer Cell 42, 1507-1527.e11. https://doi.org/10.1016/j.ccell.2024.08.012.
Ambrosetti, D., Coutts, M., Paoli, C., Durand, M., Borchiellini, D., Montemagno, C., Rastoin, O., Borderie, A., Grepin, R., Rioux-Leclercq, N., et al. (2022). Cancer-associated fibroblasts in renal cell carcinoma: implication in prognosis and resistance to anti-angiogenic therapy. BJU International 129, 80–92. https://doi.org/10.1111/bju.15506.
Klinkhammer, B.M., Goldschmeding, R., Floege, J., and Boor, P. (2017). Treatment of Renal Fibrosis—Turning Challenges into Opportunities. Advances in Chronic Kidney Disease 24, 117–129. https://doi.org/10.1053/j.ackd.2016.11.002.
Glass, D.S., Grossfeld, D., Renna, H.A., Agarwala, P., Spiegler, P., DeLeon, J., and Reiss, A.B. (2022). Idiopathic pulmonary fibrosis: Current and future treatment. The Clinical Respiratory Journal 16, 84–96. https://doi.org/10.1111/crj.13466.
Hoyer, N., Prior, T.S., Bendstrup, E., Wilcke, T., and Shaker, S.B. (2019). Risk factors for diagnostic delay in idiopathic pulmonary fibrosis. Respiratory Research 20, 103. https://doi.org/10.1186/s12931-019-1076-0.
Tomasek, J.J., Gabbiani, G., Hinz, B., Chaponnier, C., and Brown, R.A. (2002). Myofibroblasts and mechano-regulation of connective tissue remodelling. Nat Rev Mol Cell Biol 3, 349–363. https://doi.org/10.1038/nrm809.
Herrera, J., Henke, C.A., and Bitterman, P.B. (2018). Extracellular matrix as a driver of progressive fibrosis. Journal of Clinical Investigation 128, 45–53. https://doi.org/10.1172/JCI93557.
Meng, X.M., Nikolic-Paterson, D.J., and Lan, H.Y. (2016). TGF-beta: the master regulator of fibrosis. Nature Reviews Nephrology 12, 325–338. https://doi.org/10.1038/nrneph.2016.48.
Ramachandran, P., Dobie, R., Wilson-Kanamori, J.R., Dora, E.F., Henderson, B.E.P., Luu, N.T., Portman, J.R., Matchett, K.P., Brice, M., Marwick, J.A., et al. (2019). Resolving the fibrotic niche of human liver cirrhosis at single-cell level. Nature 575, 512–518. https://doi.org/10.1038/s41586-019-1631-3.
Chaudhary, N.I., Roth, G.J., Hilberg, F., Müller-Quernheim, J., Prasse, A., Zissel, G., Schnapp, A., and Park, J.E. (2007). Inhibition of PDGF, VEGF and FGF signalling attenuates fibrosis. European Respiratory Journal 29, 976–985. https://doi.org/10.1183/09031936.00152106.
Kendall, R.T., and Feghali-Bostwick, C.A. (2014). Fibroblasts in fibrosis: novel roles and mediators. Front. Pharmacol. 5. https://doi.org/10.3389/fphar.2014.00123.
Strutz, F., Zeisberg, M., Hemmerlein, B., Sattler, B., Hummel, K., Becker, V., and Müller, G.A. (2000). Basic fibroblast growth factor expression is increased in human renal fibrogenesis and may mediate autocrine fibroblast proliferation. Kidney International 57, 1521–1538. https://doi.org/10.1046/j.1523-1755.2000.00997.x.
Inoue, Y., King, T.E., Tinkle, S.S., Dockstader, K., and Newman, L.S. (1996). Human mast cell basic fibroblast growth factor in pulmonary fibrotic disorders. Am J Pathol 149, 2037–2054.
Knittel, T., Kobold, D., Piscaglia, F., Saile, B., Neubauer, K., Mehde, M., Timpl, R., and Ramadori, G. (1999). Localization of liver myofibroblasts and hepatic stellate cells in normal and diseased rat livers: distinct roles of (myo-)fibroblast subpopulations in hepatic tissue repair. Histochemistry and Cell Biology 112, 387–401. https://doi.org/10.1007/s004180050421.
Herrera, J.A., Dingle, L., Montero, M.A., Venkateswaran, R.V., Blaikley, J.F., Lawless, C., and Schwartz, M.A. (2022). The UIP/IPF fibroblastic focus is a collagen biosynthesis factory embedded in a distinct extracellular matrix. JCI Insight 7, e156115. https://doi.org/10.1172/jci.insight.156115.
Raghu, G., Remy-Jardin, M., Myers, J.L., Richeldi, L., Ryerson, C.J., Lederer, D.J., Behr, J., Cottin, V., Danoff, S.K., Morell, F., et al. (2018). Diagnosis of Idiopathic Pulmonary Fibrosis. An Official ATS/ERS/JRS/ALAT Clinical Practice Guideline. Am J Respir Crit Care Med 198, e44–e68. https://doi.org/10.1164/rccm.201807-1255ST.
Wells, A.U. (2013). Managing diagnostic procedures in idiopathic pulmonary fibrosis. European Respiratory Review 22, 158–162. https://doi.org/10.1183/09059180.00001213.
Rhim, A.D., Oberstein, P.E., Thomas, D.H., Mirek, E.T., Palermo, C.F., Sastra, S.A., Dekleva, E.N., Saunders, T., Becerra, C.P., Tattersall, I.W., et al. (2014). Stromal Elements Act to Restrain, Rather Than Support, Pancreatic Ductal Adenocarcinoma. Cancer Cell 25, 735–747. https://doi.org/10.1016/j.ccr.2014.04.021.
Sherman, M.H., Yu, R.T., Engle, D.D., Ding, N., Atkins, A.R., Tiriac, H., Collisson, E.A., Connor, F., Van Dyke, T., Kozlov, S., et al. (2014). Vitamin D Receptor-Mediated Stromal Reprogramming Suppresses Pancreatitis and Enhances Pancreatic Cancer Therapy. Cell 159, 80–93. https://doi.org/10.1016/j.cell.2014.08.007.
Egeblad, M., Ewald, A.J., Askautrud, H.A., Truitt, M.L., Welm, B.E., Bainbridge, E., Peeters, G., Krummel, M.F., and Werb, Z. (2008). Visualizing stromal cell dynamics in different tumor microenvironments by spinning disk confocal microscopy. Dis Model Mech 1, 155–167; discussion 165. https://doi.org/10.1242/dmm.000596.
Olive, K.P., Jacobetz, M.A., Davidson, C.J., Gopinathan, A., McIntyre, D., Honess, D., Madhu, B., Goldgraben, M.A., Caldwell, M.E., Allard, D., et al. (2009). Inhibition of Hedgehog Signaling Enhances Delivery of Chemotherapy in a Mouse Model of Pancreatic Cancer. Science 324, 1457–1461. https://doi.org/10.1126/science.1171362.
Zheng, Z., Peng, F., and Zhou, Y. (2024). Biomarkers in idiopathic pulmonary fibrosis: Current insight and future direction. Chinese Medical Journal Pulmonary and Critical Care Medicine 2, 72–79. https://doi.org/10.1016/j.pccm.2024.04.003.
Ley, B., Brown, K.K., and Collard, H.R. (2014). Molecular biomarkers in idiopathic pulmonary fibrosis. Am J Physiol Lung Cell Mol Physiol 307, L681–L691. https://doi.org/10.1152/ajplung.00014.2014.
Egeblad, M., Rasch, M.G., and Weaver, V.M. (2010). Dynamic interplay between the collagen scaffold and tumor evolution. Current Opinion in Cell Biology 22, 697–706. https://doi.org/10.1016/j.ceb.2010.08.015.
Astrof, S., and Hynes, R.O. (2009). Fibronectins in vascular morphogenesis. Angiogenesis 12, 165–175. https://doi.org/10.1007/s10456-009-9136-6.
Carver, W., and Goldsmith, E.C. (2013). Regulation of Tissue Fibrosis by the Biomechanical Environment. BioMed Research International 2013, 1–10. https://doi.org/10.1155/2013/101979.
Rozario, T., and DeSimone, D.W. (2010). The extracellular matrix in development and morphogenesis: A dynamic view. Developmental Biology 341, 126–140. https://doi.org/10.1016/j.ydbio.2009.10.026.
Reinke, J.M., and Sorg, H. (2012). Wound Repair and Regeneration. Eur Surg Res 49, 35–43. https://doi.org/10.1159/000339613.
Miles, F.L., and Sikes, R.A. (2014). Insidious Changes in Stromal Matrix Fuel Cancer Progression. Molecular Cancer Research 12, 297–312. https://doi.org/10.1158/1541-7786.MCR-13-0535.
Schäfer, M., and Werner, S. (2008). Cancer as an overhealing wound: an old hypothesis revisited. Nat Rev Mol Cell Biol 9, 628–638. https://doi.org/10.1038/nrm2455.
Cukierman, E., and Bassi, D.E. (2010). Physico-mechanical aspects of extracellular matrix influences on tumorigenic behaviors. Seminars in Cancer Biology 20, 139–145. https://doi.org/10.1016/j.semcancer.2010.04.004.
Midwood, K.S., Williams, L.V., and Schwarzbauer, J.E. (2004). Tissue repair and the dynamics of the extracellular matrix. The International Journal of Biochemistry & Cell Biology 36, 1031–1037. https://doi.org/10.1016/j.biocel.2003.12.003.
McDonald, J.A., Kelley, D.G., and Broekelmann, T.J. (1982). Role of fibronectin in collagen deposition: Fab’ to the gelatin-binding domain of fibronectin inhibits both fibronectin and collagen organization in fibroblast extracellular matrix. The Journal of cell biology 92, 485–492. https://doi.org/10.1083/jcb.92.2.485.
Kadler, K.E., Hill, A., and Canty-Laird, E.G. (2008). Collagen fibrillogenesis: fibronectin, integrins, and minor collagens as organizers and nucleators. Current Opinion in Cell Biology 20, 495–501. https://doi.org/10.1016/j.ceb.2008.06.008.
Dzamba, B.J., Wu, H., Jaenisch, R., and Peters, D.M. (1993). Fibronectin binding site in type I collagen regulates fibronectin fibril formation. The Journal of cell biology 121, 1165–1172. https://doi.org/10.1083/jcb.121.5.1165.
McDonald, J.A., Quade, B.J., Broekelmann, T.J., LaChance, R., Forsman, K., Hasegawa, E., and Akiyama, S. (1987). Fibronectin’s cell-adhesive domain and an amino-terminal matrix assembly domain participate in its assembly into fibroblast pericellular matrix. Journal of Biological Chemistry 262, 2957–2967. https://doi.org/10.1016/S0021-9258(18)61453-X.
Li, S., Van Den Diepstraten, C., D’Souza, S.J., Chan, B.M.C., and Pickering, J.G. (2003). Vascular smooth muscle cells orchestrate the assembly of type I collagen via alpha2beta1 integrin, RhoA, and fibronectin polymerization. Am J Pathol 163, 1045–1056. https://doi.org/10.1016/s0002-9440(10)63464-5.
Filla, M.S., Dimeo, K.D., Tong, T., and Peters, D.M. (2017). Disruption of fibronectin matrix affects type IV collagen, fibrillin and laminin deposition into extracellular matrix of human trabecular meshwork (HTM) cells. Experimental Eye Research 165, 7–19. https://doi.org/10.1016/j.exer.2017.08.017.
Sottile, J., Shi, F., Rublyevska, I., Chiang, H.-Y., Lust, J., and Chandler, J. (2007). Fibronectin-dependent collagen I deposition modulates the cell response to fibronectin. American Journal of Physiology-Cell Physiology 293, C1934–C1946. https://doi.org/10.1152/ajpcell.00130.2007.
Sottile, J., and Hocking, D.C. (2002). Fibronectin Polymerization Regulates the Composition and Stability of Extracellular Matrix Fibrils and Cell-Matrix Adhesions. MBoC 13, 3546–3559. https://doi.org/10.1091/mbc.e02-01-0048.
Altrock, E., Sens, C., Wuerfel, C., Vasel, M., Kawelke, N., Dooley, S., Sottile, J., and Nakchbandi, I.A. (2015). Inhibition of fibronectin deposition improves experimental liver fibrosis. Journal of Hepatology 62, 625–633. https://doi.org/10.1016/j.jhep.2014.06.010.
Antia, M., Baneyx, G., Kubow, K.E., and Vogel, V. (2008). Fibronectin in aging extracellular matrix fibrils is progressively unfolded by cells and elicits an enhanced rigidity response. Faraday Discuss. 139, 229. https://doi.org/10.1039/b718714a.
Sottile, J., Schwarzbauer, J., Selegue, J., and Mosher, D.F. (1991). Five type I modules of fibronectin form a functional unit that binds to fibroblasts and Staphylococcus aureus. Journal of Biological Chemistry 266, 12840–12843. https://doi.org/10.1016/S0021-9258(18)98769-7.
Peake, P., Gooley, A., and Britton, W.J. (1993). Mechanism of interaction of the 85B secreted protein of Mycobacterium bovis with fibronectin. Infect Immun 61, 4828–4834. https://doi.org/10.1128/iai.61.11.4828-4834.1993.
Lindmark, H., and Guss, B. (1999). SFS, a Novel Fibronectin-Binding Protein from Streptococcus equi , Inhibits the Binding between Fibronectin and Collagen. Infect Immun 67, 2383–2388. https://doi.org/10.1128/IAI.67.5.2383-2388.1999.
Joh, D., Wann, E.R., Kreikemeyer, B., Speziale, P., and Höök, M. (1999). Role of fibronectin-binding MSCRAMMs in bacterial adherence and entry into mammalian cells. Matrix Biology 18, 211–223. https://doi.org/10.1016/S0945-053X(99)00025-6.
Schwarz-Linek, U., Gurusiddappa, S., Kim, J.H., Pilka, E.S., Briggs, J.A.G., Gough, T.S., Campbell, I.D., and Potts, J.R. (2003). Pathogenic bacteria attach to human fibronectin through a tandem b-zipper. Nature 423.
Arnoldini, S., Moscaroli, A., Chabria, M., Hilbert, M., Hertig, S., Schibli, R., Béhé, M., and Vogel, V. (2017). Novel peptide probes to assess the tensional state of fibronectin fibers in cancer. Nat Commun 8, 1793. https://doi.org/10.1038/s41467-017-01846-0.
Chabria, M., Hertig, S., Smith, M.L., and Vogel, V. (2010). Stretching fibronectin fibres disrupts binding of bacterial adhesins by physically destroying an epitope. Nat Commun 1, 135. https://doi.org/10.1038/ncomms1135.
Tomasini-Johansson, B.R., Kaufman, N.R., Ensenberger, M.G., Ozeri, V., Hanski, E., and Mosher, D.F. (2001). A 49-Residue Peptide from Adhesin F1 of Streptococcus pyogenes Inhibits Fibronectin Matrix Assembly. Journal of Biological Chemistry 276, 23430–23439. https://doi.org/10.1074/jbc.M103467200.
Tomasini-Johansson, B.R., Zbyszynski, P.W., Toraason, I., Peters, D.M., and Kwon, G.S. (2018). PEGylated pUR4/FUD peptide inhibitor of fibronectin fibrillogenesis decreases fibrosis in murine Unilateral Ureteral Obstruction model of kidney disease. PLOS ONE 13, e0205360. https://doi.org/10.1371/journal.pone.0205360.
Zbyszynski, P., Tomasini-Johansson, B.R., Peters, D.M., and Kwon, G.S. (2018). Characterization of the PEGylated Functional Upstream Domain Peptide (PEG-FUD): a Potent Fibronectin Assembly Inhibitor with Potential as an Anti-Fibrotic Therapeutic. Pharm Res 35, 126. https://doi.org/10.1007/s11095-018-2412-7.
Zbyszynski, P., Toraason, I., Repp, L., and Kwon, G.S. (2019). Probing the subcutaneous absorption of a PEGylated FUD peptide nanomedicine via in vivo fluorescence imaging. Nano Convergence 6, 22. https://doi.org/10.1186/s40580-019-0192-3.
Lee, H.J., Bernau, K., Harr, T.J., Rosenkrans, Z.T., Kessler, G.A., Stott, K., Oler, A.T., Rahar, B., Zhu, T., Medina-Guevara, Y., et al. (2024). [64Cu]Cu-PEG-FUD peptide for noninvasive and sensitive detection of murine pulmonary fibrosis. Science Advances 10, eadj1444. https://doi.org/10.1126/sciadv.adj1444.
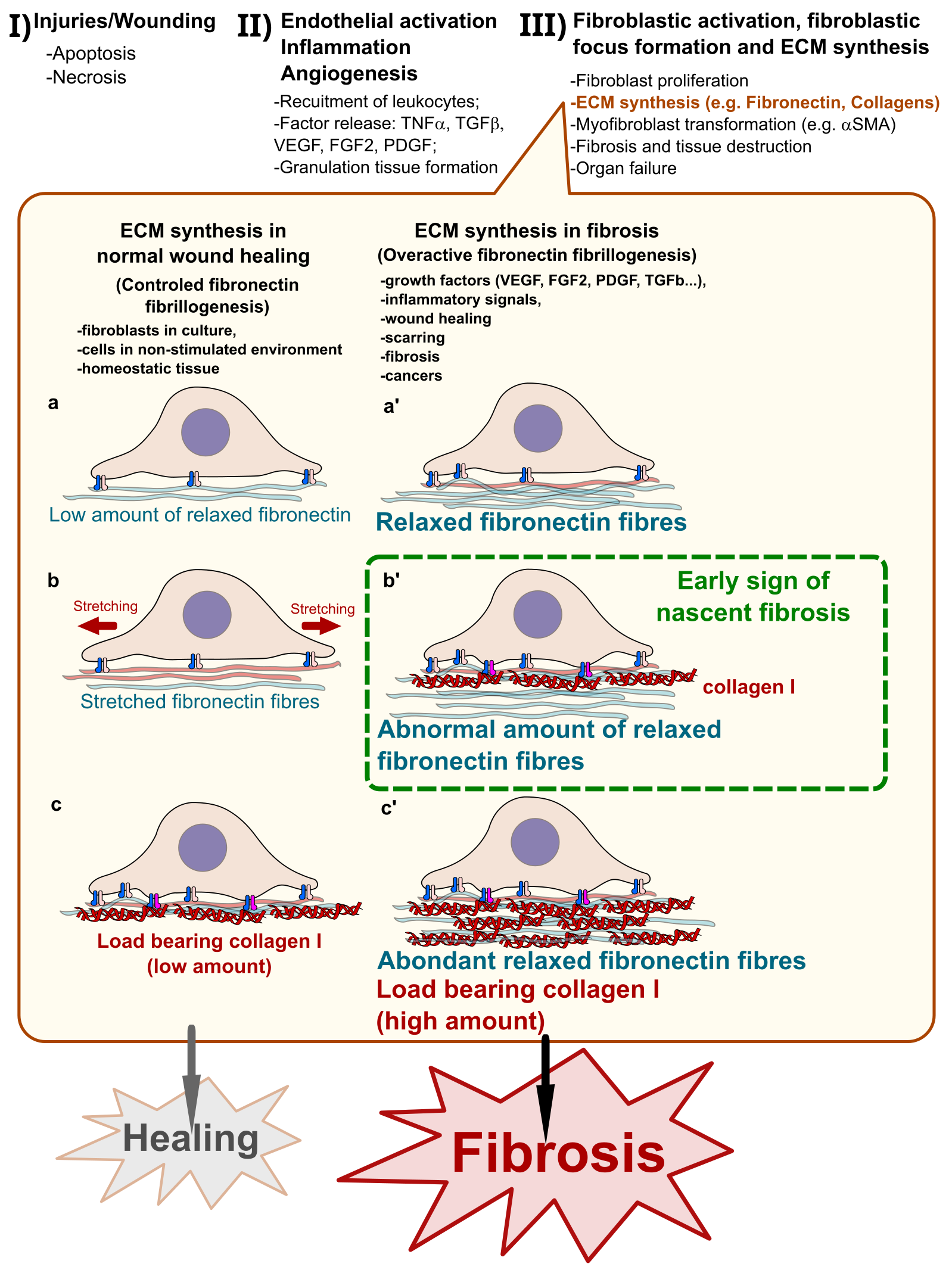
Downloads
Published
Issue
Section
Categories
License
Copyright (c) 2024 Dr Adama Sidibé (Author)

This work is licensed under a Creative Commons Attribution 4.0 International License.
Copyright © 2024 The author, Rviews Press Marseille, France. All right reserved including those for text, images, AI training and AI-like technologies. The articles published in Cell Biology are distributed under the Creative Commons Attribution 4.0 International (CC BY)